Unfolding cellular mechanisms by Ca2+ signaling-based research
Ca2+ signaling is one of the most important signaling mechanisms regulating myriads of cellular functions (Fig. 1). We have identified basic mechanisms of the diverse spatiotemporal dynamics of Intracellular Ca2+ signals, which underlie the versatility of Ca2+ in cell signaling. Based on these key findings, we are aiming at deciphering physiological and pathophysiological functions of Ca2+ at all levels spanning from the molecules to whole body.
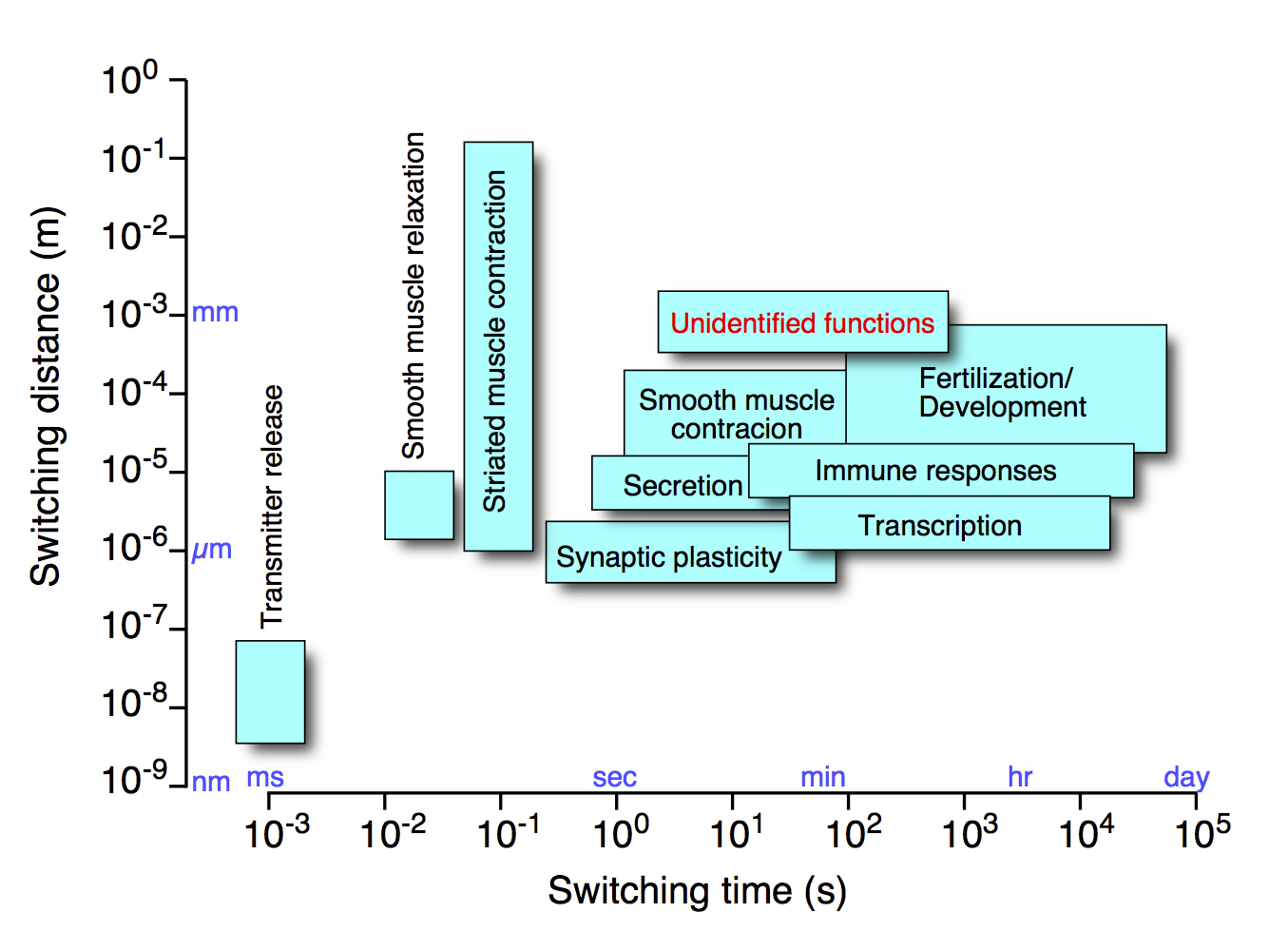
1. BASIC MECHNISMS OF Ca2+ RELEASE
While Ca2+ signal can be generated by an influx of Ca2+ from the extracellular space, Ca2+release from the intracellular store also plays important roles in generation of Ca2+ signals. The endoplasmic reticulum functions as the major intracellular Ca2+ store, and Ca2+ can be released via two types of Ca2+ release channel; ryanodine receptors and inositol 1,4,5-trisphosphate (IP3) receptors (Fig. 2).
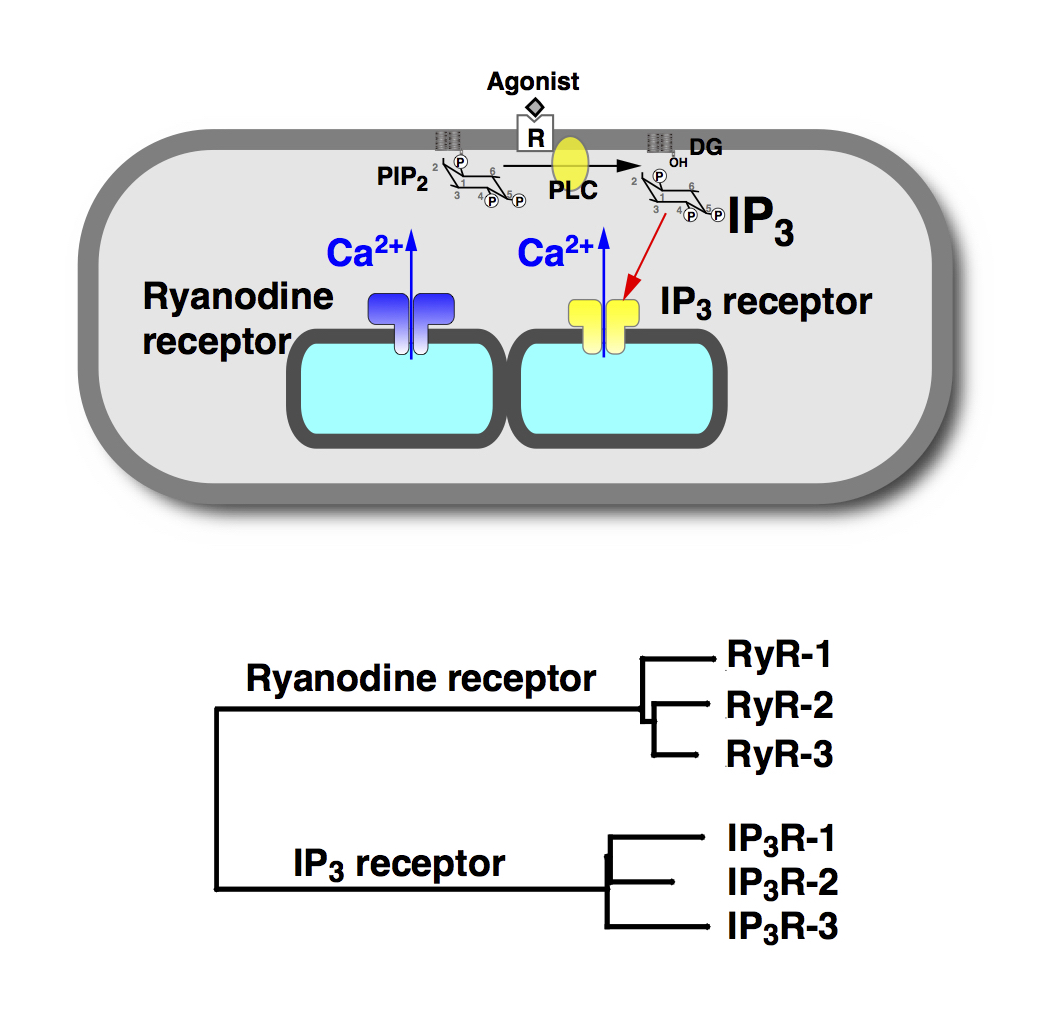
Our laboratory has clarified basic mechanisms of the Ca2+ release and Ca2+ signaling mechanisms as summarized below.
Ca2+ signals show very dynamic spatiotemporal changes. This property allows the Ca2+ signal to be an extremely versatile cellular switch regulating diverse cell functions (Fig. 1). One of the most notable spatiotemporal patterns of Ca2+ signals is a wave-like increase in intracellular cytoplasmic Ca2+ concentration ([Ca2+]Cyt), or Ca2+ wave. Another remarkable spatiotemporal pattern is an oscillatory change in [Ca2+]Cyt, or Ca2+ oscillation. Indeed, many cellular functions are regulated by the frequency of Ca2+ oscillation. However, fundamental questions remain. How and what for does [Ca2+]Cyt oscillate? We addressed these important questions.
First, we studied IP3-induced Ca2+ release mechanism, which is one of the most important Ca2+ mobilizing mechanisms in many types of cell. We showed that activation of the IP3 receptor (IP3R) requires not only IP3 but also Ca2+ (J. Gen. Physiol., 1990; Nature, 1992). Therefore, Ca2+release via the IP3R is under the positive feedback control of Ca2+ that is released through the IP3R. The positive feedback loop makes Ca2+ release via IP3R regenerative. This property nicely explains the reason for the generation of Ca2+ wave (Fig. 3).
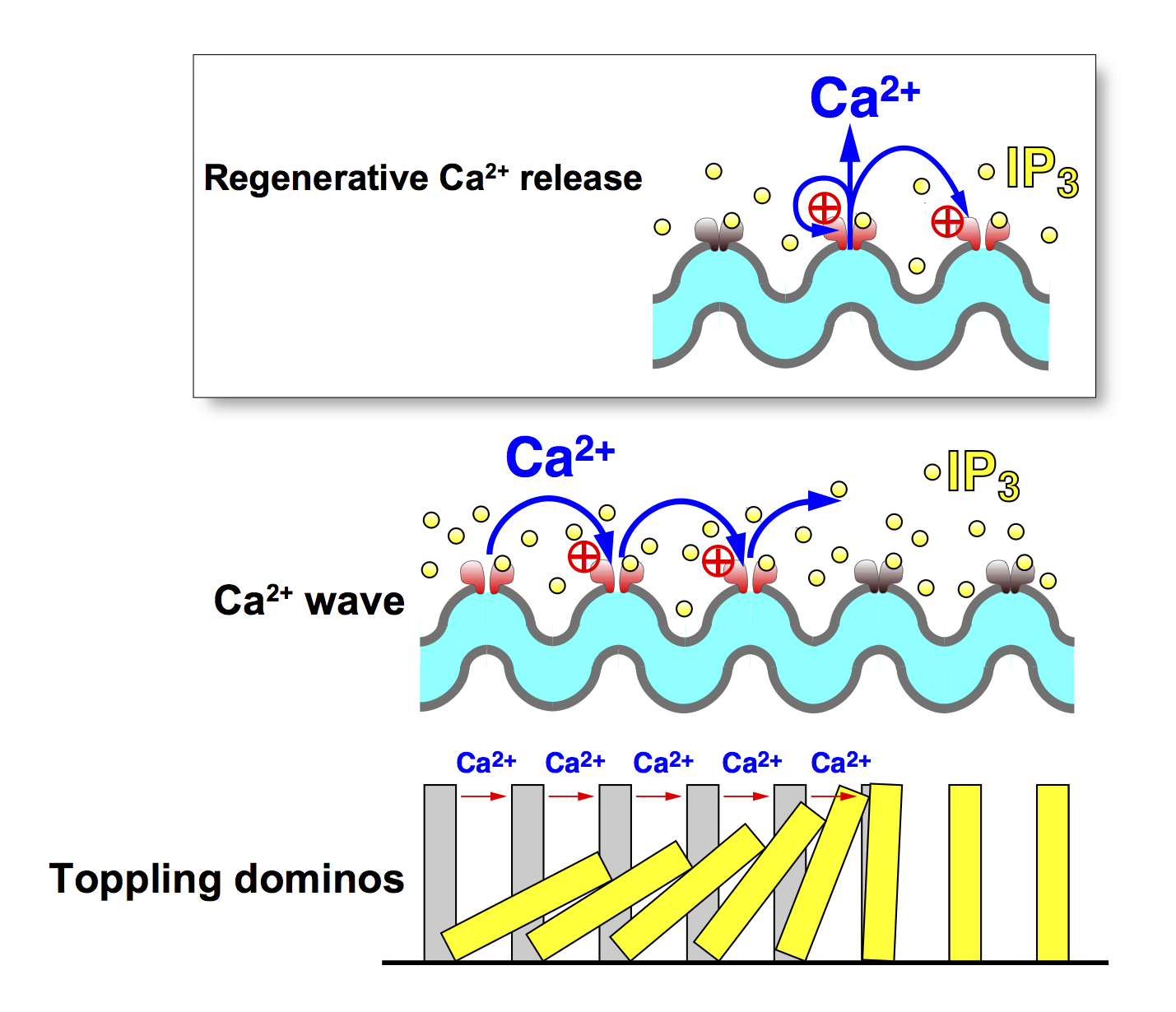
There are three subtypes of IP3R, which have different sensitivities to IP3 and Ca2+ concentrations. We showed that these subtypes generate different patterns of Ca2+ oscillation (EMBO J. 1999). We then identified the Ca2+ sensor region of the IP3R, and experimentally showed that the positive feedback regulation of IP3R via the Ca2+ sensor of IP3R indeed plays an essential role in regulating Ca2+ oscillations (EMBO J., 2001). Thus, our results clarified that the regeneratively of IP3-induced Ca2+ release is fundamental to the generation of Ca2+ oscillation.
What for, then, does [Ca2+]Cyt oscillate? We addressed this question looking at transcription by the nuclear factor of activated T cells (NFAT), which is one of the important cellular functions that are regulated by the Ca2+ oscillation frequency. NFAT is dephosphorylated by Ca2+-dependent phosphatase, calcineurin. Upon dephosphorylation, NFAT is translocated from the cytoplasm to the nucleus to initiate transcription. We analyzed the kinetics of the dephosphorylation and translocation of NFAT, and found that the dephosphorylated form of NFAT functions as a working memory of transient increases in [Ca2+]Cyt (EMBO J. 2003). With increasing frequency of Ca2+ oscillation, dephosphorylated NFAT accumulates in the cytoplasm to enhance its nuclear translocation. This is the molecular basis of the mechanism that decodes the Ca2+ oscillation frequency. We further showed that Ca2+oscillation is more cost-effective in regulating cell functions than a continuous increase in Ca2+ (EMBO J. 2003). These studies provide us with an insight into the reason why [Ca2+]Cyt has to oscillate.
- Iino, M. J. Gen. Physiol. 94, 363–383, 1989.
- Iino, M. J. Gen. Physiol. 95, 1103–1122, 1990.
- Iino, M. J. Gen. Physiol. 98, 681–698, 1991.
- Iino, M. & Endo, M. Nature 360, 76–78, 1992.
- Iino, M. et al. EMBO J. 12, 5287-5291, 1993.
- Iino, M. et al. EMBO J. 13, 5026-5031, 1994.
- Hirose, K. & Iino, M. Nature 372, 791–794, 1994.
- Takeshima, H. et al. Nature 369, 556–559, 1994.
- Takeshima, H. et al. EMBO J. 14, 2999–3006, 1995.
- Takeshima, H. et al. J. Biol. Chem. 271, 19649–19652, 1996.
- Yamazawa, T. et al. EMBO J. 15, 6172–6177, 1996.
- Takeshima, H. et al. EMBO J. 17, 3309–3316, 1998.
- Miyakawa, T. et al. EMBO J. 18, 1303–1308, 1999.
- Miyakawa, T. et al. EMBO J. 20, 1674-1680, 2001.
- Tomida, T. et al. EMBO J. 22, 3825–3832, 2003.
2. IMAGING STUDIES
Our study on Ca2+ signaling made us realize the importance of visualization of signaling molecules within living cells. Thus, our laboratory has been involved in the generation of new indicators of signaling molecules upstream and downstream Ca2+ signals.
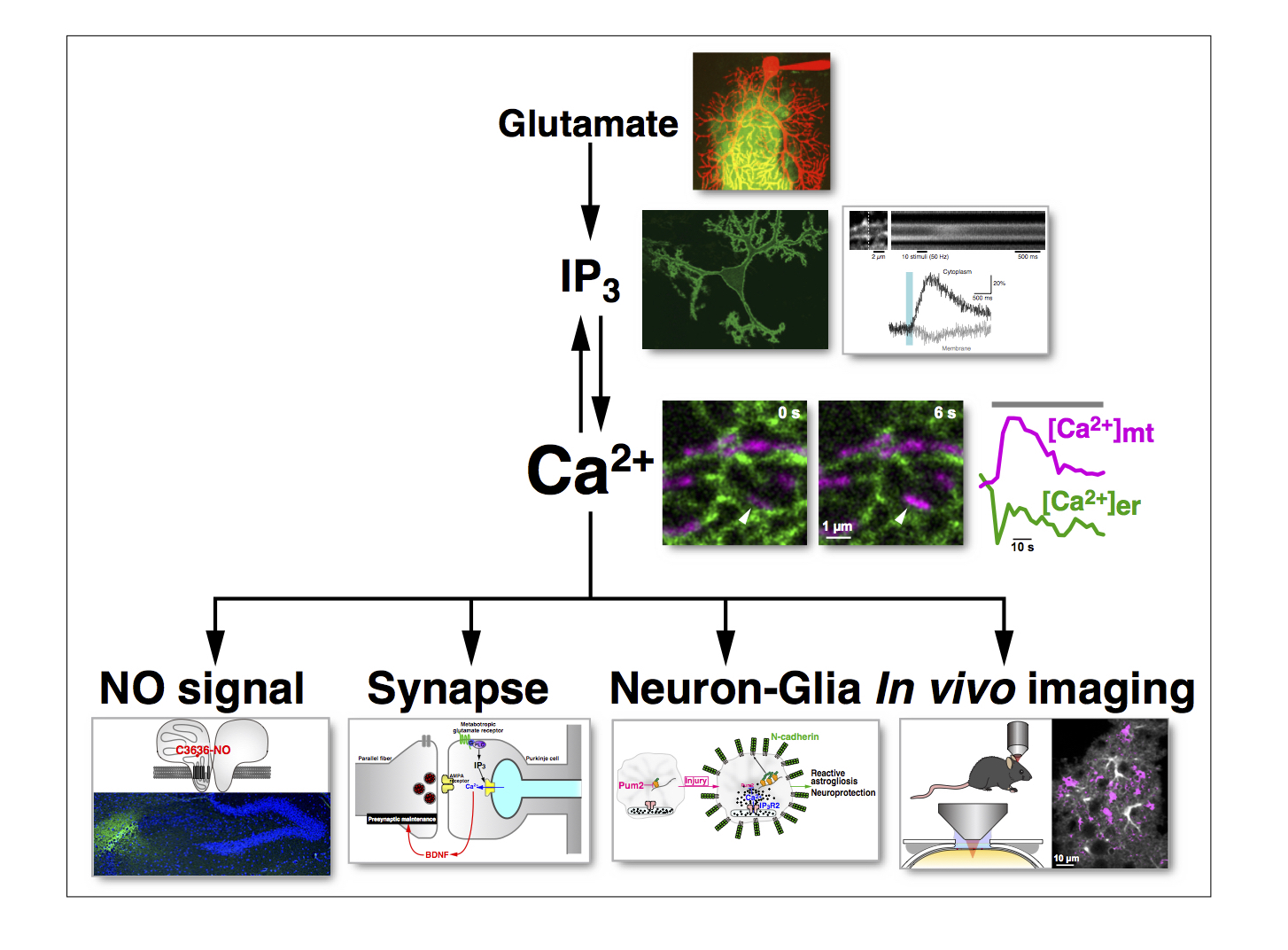
IP3 imaging
We generated an IP3 indicator based on the PH domain of PLC{delta}1, and have succeeded in imaging IP3 signaling in various cells including intact neurons within cerebellar slice preparations. Our study showed that IP3 signaling in cerebellar Purkinje cells are cooperatively regulated by both metabotropic and ionotropic glutamate receptors (Fig. 5).
- Hirose, K. et al. Science 284, 1527–1530, 1999.
- Okubo, Y. et al. Neuron 32, 113–122, 2001.
- Okubo, Y. et al. J. Neurosci. 24, 9513–9520, 2004.
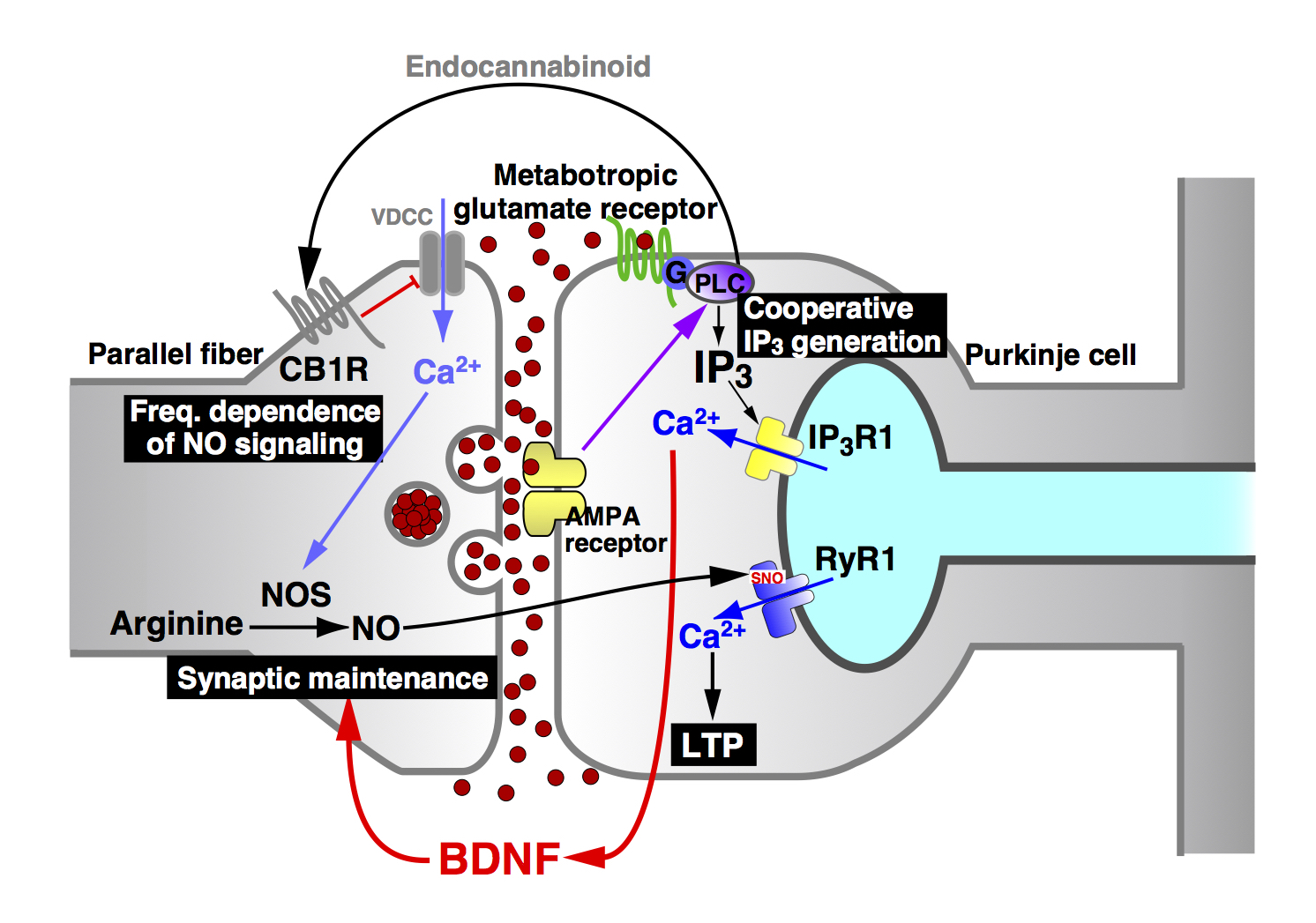
Nitric oxide (NO) imaging
We generated a nitric oxide (NO) indicator, HBR-GFP, based on the heme-binding region of soluble guanylyl cyclase (the physiological target of NO). This indicator was successfully used in cerebellar slice preparations to image NO signals in response to parallel fiber (PF) stimulation. We found that the NO signal intensity decreases steeply with distance from the activated synapse, and NO generates synapse-specific long-term potentiation (LTP) of PF-Purkinje cell synapses. We also showed that the NO signal intensity depends biphasically on the frequency of PF stimulation. (This means that there is an optimal firing frequency for NO generation.) Importantly, the LTP depends similarly on the frequency of PF stimulation (Fig. 5). Thus, our NO indicator provided us with valuable information regarding the role of NO signals in the central nervous system.
- Namiki, S. et al. J. Physiol., 566, 849–863, 2005.
Glutamate imaging
Glutamate is the most important excitatory neurotransmitter in the brain. We generated a new glutamate indicator (EOS) based on the glutamate binding site of the ionotropic glutamate receptor (GluR2). We have successfully used this indicator to image glutamate dynamics in acute brain slices and in cerebral cortex in vivo (Fig. 5).
- Namiki, S. et al. Eur. J. Neurosci. 25, 2249–2259, 2007.
- Okubo, Y. et al. Proc. Nat. Acad. Sci. U.S.A. 107, 6526–6531, 2010.
Intraorganellar Ca2+ imaging
As described above, Ca2+ is released from the ER. Therefore, Ca2+ concentration within the ER ([Ca2+]ER) is an important information. However, [Ca2+]ER) is several thousand-fold higher than that of [Ca2+]Cyt, and It has been difficult to measure [Ca2+]ER with a high signal-to-noise ratio. Based on a genetically encoded Ca2+ indicator, we successfully generated intraorganellar Ca2+ indicators (CEPIAs) that are optimized for measuring [Ca2+]ER. We also generated variants of CEPIA that are suitable for measuring Ca2+ in mitochondria ([Ca2+]Mit). Since we generated CEPIAs with three different fluorescence colors, we can now measure [Ca2+]Cyt, [Ca2+]ER, and [Ca2+]Mit, simultaneously. Using CEPIA we have succeeded in imaging ER Ca2+ dynamics in postsynaptic neurons in the cerebellum. We observed synaptic input-dependent Ca2+ release from the ER. Furthermore, we observed activity-dependent accumulation of Ca2+ in the ER, so that ER functions as a memory of nervous activities.
- Ishii, K., et al. EMBO Rep. 7, 390–396, 2006.
- Suzuki, J. et al. Nat. Commun. 5:4153, 2014.
- Okubo, Y. et al. J. Neurosci. 35, 15837–15846, 2015.
3. SEARCH FOR NEW ROLES OF Ca2+ AND RELATED SIGNALING MOLECULES
Our laboratory has been trying to identify hitherto unrecognized functions of Ca2+ signals especially in the brain in both health and disease. We have a very powerful tool to study the role of Ca2+ signals, i.e., the IP3 hydrolyzing enzyme, IP3 5-phosphatase, which effectively shuts down IP3 signaling. Indeed, in many cell types expression of the enzyme virtually abolishes agonist-induced Ca2+ release.
Physiological functions
Using the method to inhibit IP3-induced Ca2+ release, we have so far identified an activity-dependent synaptic maintenance mechanism in the cerebellar cortex (Fig. 5) and a neuron-astrocyte interaction in terms of the promotion of neurite growth.
We also studied the role of NO signaling in the brain. We showed that NO induces Ca2+ release from the intracellular sore in cerebellar Purkinje cells. Our in-depth analyses showed that NO S-nitrosylates the type 1 ryanodine receptor (RyR1) at a specific cysteine residue (Cys3636 in mouse, Cys3635 in human) to induce activation of the Ca2+ release channel. This NO-induced Ca2+ release (NICR) leads to long-term potentiation (LTP) of the parallel fiber-Purkinje cell synapse (Fig. 5).
Pathophysiological functions
We then generated a knock-in mouse line, in which the important cysteine residue (Cys3636) is replaced with alanine so that NICR is deficient in these animals. Interestingly, we found that NICR also has a pathophysiological role in neuronal injury during hyperactivity of neurons (excitotoxicity). In NICR-deficient mice, seizure-induced neuronal injury was significantly ameliorated. Furthermore, we found that a pharmacological agent, dantrolene, which inhibits NICR has a neuroprotective effect. Thus, NICR is involved in excitotoxic neuron injury (Fig. 6), and inhibition of NICR may have a therapeutic value for excitotoxicity.
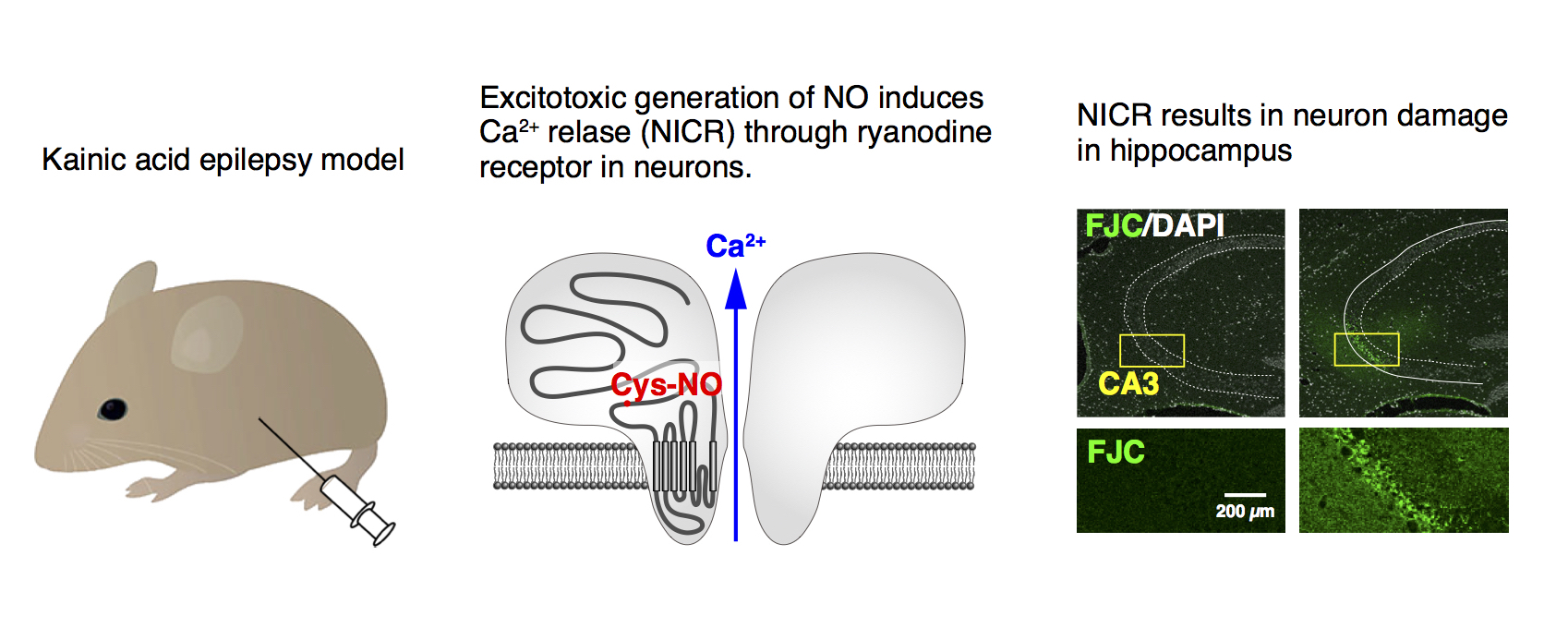
Our in vivo Ca2+ imaging in astrocytes showed that these cells generate robust Ca2+ oscillations after brain injury. These Ca2+ oscillations are absent in IP3R2-KO mice. By comparing the effect of brain injury between WT and IP3R2-KO mice, we found that astrocytic Ca2+ oscillations are required for reactive astrogliosis and neuroprotection (Fig. 7).
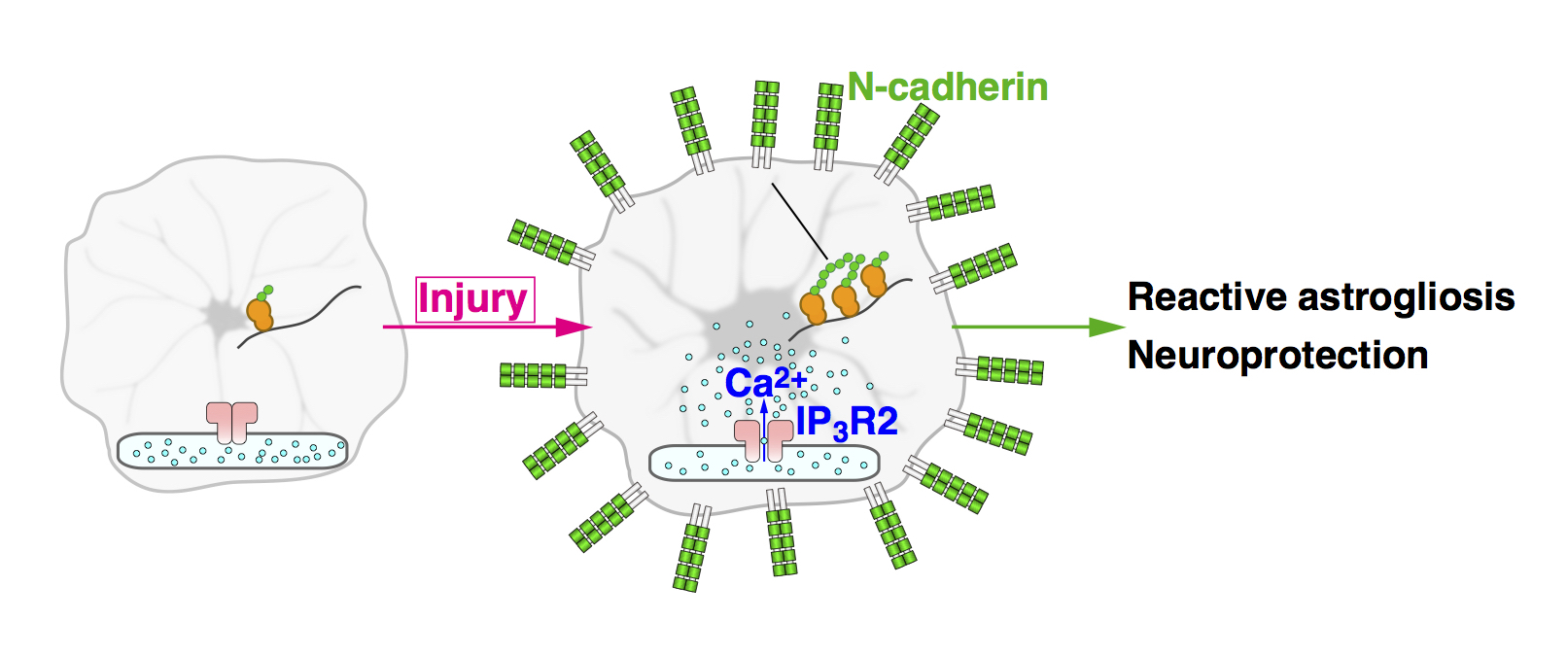
- Furutani, K. et al. Proc. Nat. Acad. Sci. U.S.A. 103, 8528–8533, 2006.
- Kanemaru, K. et al. J. Neurosci. 27, 8957–8966, 2007.
- Mashimo, M. et al. Eur. J. Neurosci. 32, 1668–1677, 2010.
- Kakizawa, S. et al. EMBO J. 31, 417–428, 2012.
- Kanemaru, K. et al. Proc. Nat. Acad. Sci. U.S.A. 110, 11612–11617, 2013.
- Ino, D. et al. Cell Rep. 2015.
- Mikami, Y. et al. EBioMedicine. 11, 253–261, 2016.
4. IN VIVO Ca2+ IMAGING
Our goal of Ca2+ imaging is to image Ca2+ signals (and other signaling molecules) within individual cells of a living animal. We have succeeded in imaging Ca2+ signals and other signaling molecules such as glutamate in the brain of living mice (Fig. 8).
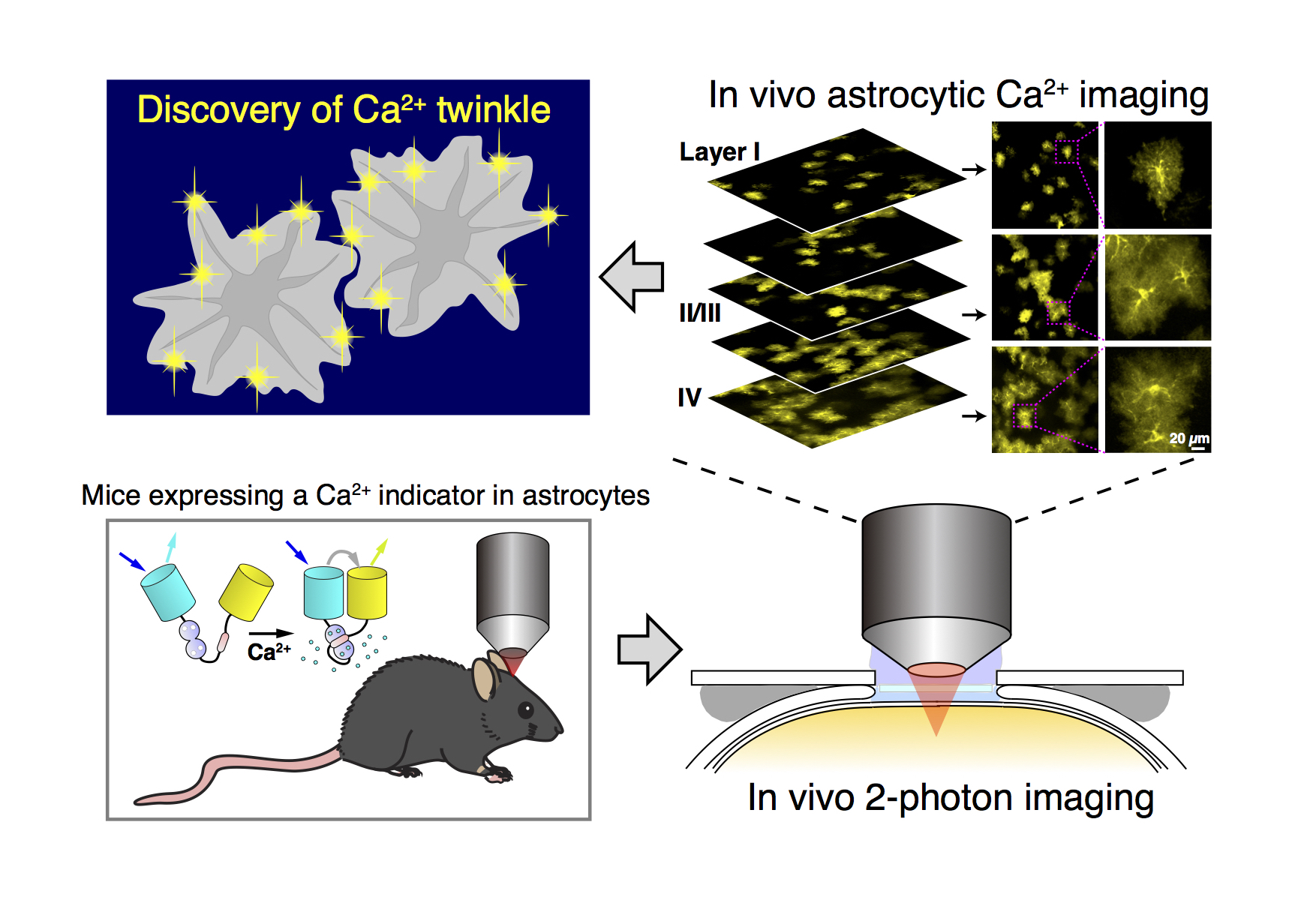
We are now trying to expand the list of organs for in vivo imaging.
- Okubo, Y. et al. Proc. Nat. Acad. Sci. U.S.A. 107, 6526–6531, 2010.
- Kanemaru, K. et al. Cell Rep. 2014.
5. FUTURE DIRECTIONS
Combining new imaging tools and the specific method to inhibit Ca2+ signals, we wish to contribute to the advancement of our knowledge in cell signaling especially in conjunction with brain functions.